Water, water, everywhere.
Dr. Leigh Fletcher appeared on this month’s episode of BBC Sky at Night to discuss Juno’s goals at Jupiter, and describes the importance of Jupiter’s water in this new post.
If our ideas about the formation of giant planets stand up to the observational tests of the Juno spacecraft, then Jupiter’s extensive atmosphere should be moist, humid and drenched in water. Moist air contains energy, released as gaseous water condenses to liquid droplets. That energy may be powering the fascinating meteorology that shapes the face of the giant planet, and driving lighting storms that flicker and flash in Jupiter’s belts. In turn, the distribution of water may help to explain the contrasts in storm activity and colouration between the white zones and brown belts that criss-cross the face of Jupiter. Understanding Jupiter’s water might be the key to understanding its churning weather.
But if the distribution and availability of water is key to explaining the meteorology of Jupiter, then why don’t we already have a better handle on this question? The answer lies in Jupiter’s cold atmospheric temperatures. The topmost clouds that we can see through our telescopes are composed of crystals of ammonia ice, mixed with various chemical contaminants that cause the different cloud colours. These condense at a lower temperature (roughly -100 degree Celsius) than water vapour, so the clouds of ammonia ice sit higher up than the water clouds. In fact, this cloud layer almost completely hides the deeper layers from view, and we have only glimpsed water ice in very limited locales under very special circumstances, when a powerful storm dredges the water ices upwards to the levels where we can see it, before precipitation (as rain or snow) causes it to sink back down again.
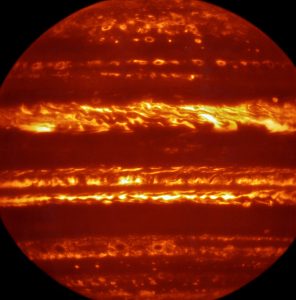
Jupiter in infrared light – spectra of the brightest regions show some signs of the presence of water, but cannot map the deep, drenched interior. Credit: ESO/L.N. Fletcher
Certain wavelengths of light can start to probe down through these topmost cloud layers, like removing the skin of an onion to see what lies beneath. For example, Earth-based telescopes can use observations of Jupiter’s infrared glow at 5 microns to peer through gaps in the ammonia ice clouds, such as those recently released by the Very Large Telescope in Chile. Previous spacecraft, such as Galileo and Cassini, have had instruments that can observe Jupiter in this spectral `window’, and allowed us to place lower limits on the amount of water present (around 0.04% by volume). However, they never sense down deep enough to see the amount of water in the deeper atmosphere, which is expected to be in the 0.1-1.0% range, depending on the different models used. Juno carries a similar infrared instrument called JIRAM, provided by the Italian Space Agency, which will perform similar measurements. But the deep water abundance remains out of reach for these infrared mappers.
A much better solution, then, is to actually send a probe into Jupiter itself. And that’s precisely what we did in 1995 with the Galileo probe, which carried all the sensors required to sniff out the gases and clouds in Jupiter’s cloudy layers. However, with only one probe there’s always the risk that you’ll find a region that isn’t representative of the full planet. Imagine trying to understand the amount of water on Earth if you only sampled the Sahara desert. The probe descended into a location known as a ‘hotspot’ just north of the equator, where powerful downwelling regions dried the atmosphere out and removed almost all traces of water. The probe transmission ended at 20 bar of pressure, below the expected altitude of the water cloud (around 5-7 bar), but still without finding the deep abundance. The maximum was 490 ppm near 20 bar (0.05% by volume), around 30% of the solar abundance. Based on Galileo’s measurements of other species, like methane and ammonia, we’d expect that value to be more like 400-1000% of the solar abundance.
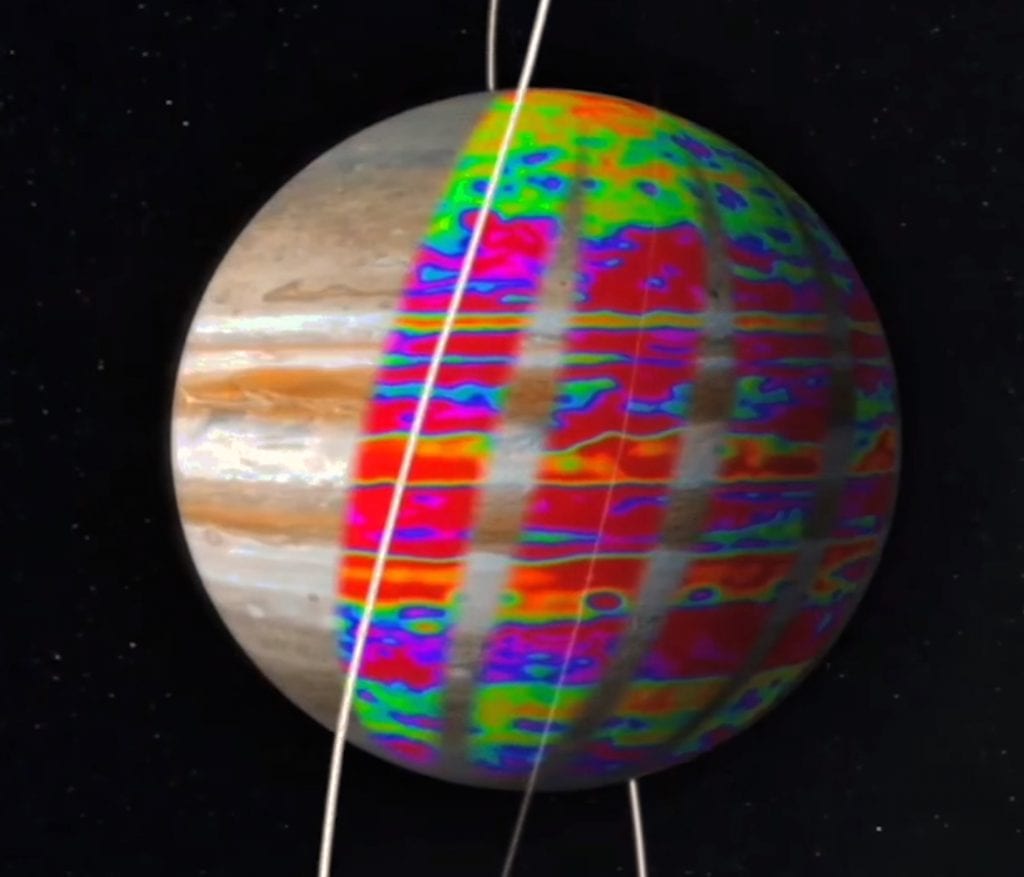
Mapping of Jupiter’s deep water and ammonia, key condensible species, far below the obscuring clouds. Credit: NASA
It’s this mystery that’s driving Juno, and its microwave radiometer experiment. Microwave light is another way of peering below the topmost clouds, and this should give us access down to 100 bar of pressure to see the distribution of water down at great depths. We’ll be going deeper into the churning, convective weather layer than ever before to understand not only the bulk abundance of water, but also its distribution. Maybe there’s more water available beneath the belts to power the moist convection and lightning storms that we see there? But it’s a balancing act, and too much water actually stabilises the atmosphere and prevents convection – maybe that’s what’s happening beneath the white zones, where we don’t see as much convection? Or is water really depleted throughout the cloud forming region, as the Galileo probe results suggested? Juno is about to provide the answer.
At the same time, we’ll be amassing data from Earth that reveals the temperature, cloud and compositional structure above the clouds to see how this relates back to the distribution of water far below. Will the deep atmospheric dynamics be different to what we’re used to above Jupiter’s clouds? We hope to finally have a handle on the ‘Jovian water cycle’ that powers the weather on this gas giant world.
Comments are closed, but trackbacks and pingbacks are open.